Raman spectroscopy is utilized in a variety of ways, ranging from the characterization of protein-nucleic acid interactions1 to analyzing dyes in ancient artwork2. When dealing with sensitive samples, a common issue one runs into is damaging the sample. Laser powers for Raman systems can have a very large range, and the initial thought is that if you turn the power down, you can eliminate sample damage. While this is true to some extent, it is certainly not the whole story. In order to maximize signal while minimizing or eliminating sample damage all together, one must consider the following:
Laser source:
Raman laser sources are typically chosen to be continuous wave (CW) in the milliwatt range, although there are some studies that use ultrafast pulsed laser sources which have peak powers on the order of 1 billion watts! Since the Raman signal is directly proportional to the input laser power, the signal can be amplified by ramping up the power of the incident laser beam. This may seem to indicate that the best choice is an ultrafast pulsed laser, but there are several reasons why this is not the case:
- Ultrafast laser systems are incredibly expensive, costing several hundred thousand dollars.
- One of the major benefits of Raman spectroscopy is the ability to conduct very high resolution measurements. Because the large spectral bandwidth imposed on a pulsed laser by the uncertainty principle, high resolution measurements can be very difficult to obtain.
- Pulsed lasers typically run with a low repetition rate (usually around 1 kHz – 5 kHz), meaning that most of the time, no light is hitting the sample. Since the incident laser is effectively “off” the majority of the time, the amount of Raman photons being generated is not as much as one might initially think.
- When a pulse does hit the sample, it is doing so with up to 1 billion watts. Such high power is almost certain to damage the sample in all but a select few cases.
By contrast, a continuous wave laser source holds many benefits over the ultrafast systems.
- CW laser sources are much more compact and inexpensive, as well as less sensitive to environmental variations (temperature, humidity, vibrations, etc.). View StellarNet Raman Lasers.
- CW lasers have a very narrow spectral bandwidth (<10 MHz), allowing for very high resolution measurements. In fact, when using a CW laser source, we are typically limited by the detector resolution rather than the source. StellarNet offers these Raman detectors with sub-nm resolution. View Raman Spectrometers.
- Continuous wave lasers are just that – continuous. Because they are not pulsed, photons are hitting the sample at all times (i.e. the laser is always “on”).
- CW laser powers typically range anywhere from 1 mW – 500 mW – considerably lower power than ultrafast sources.
***Because most Raman spectroscopists are using CW laser sources, the rest of this article will ignore sample damage concerns with ultrafast laser systems and focus only on CW systems.
Input wavelength:
As we all know, Raman spectroscopy (and all spectroscopies for that matter) rely on quantum effects. As such, we can’t just consider the laser power, we must also account for the laser wavelength. The most common laser wavelengths used in Raman spectroscopy are 532 nm, 785 nm, and 1064 nm. At longer wavelengths we typically see more laser absorption which results in sample heating. This heating can lead to alterations in experimental conditions (i.e. the vibrational spectrum of a sample at different temperatures can be drastically different) and even sample damage (liquid samples may boil and darkly colored samples can burn or even ignite). It is important to choose your laser wavelength carefully depending on what sample you are probing. While typically speaking, a 532 nm laser will be absorbed less by the sample, one can imagine a specific sample where 532 nm laser light can actually ionize the sample, effectively destroying it. Additionally, we often see heating occur when the input wavelength coincides with an absorption band from the sample wherein the relaxation pathway generates a great deal of heat. In both of these cases, it may make sense to use a different wavelength source (specifically a longer wavelength for the former, whereas the latter may simply need to change by a few nm in either direction).
Sample:
The nature of the sample itself will go a long way in determining what laser power and wavelength you will need. One must consider any absorption bands or band-gaps that may exist, and how excitation to those bands affects the sample from then on. Of course, sometimes you may be working with an unknown sample, and so you don’t know exactly where to expect an absorption band. In that case, think of the color of that sample. If it is darkly colored, it will absorb most colors of light and be highly likely to melt, burn, or ignite. To avoid this, consider using UV or NIR wavelengths.
Indications of sample damage:
There are some obvious indications you are damaging the sample;
- You can physically see the damage – i.e. you can see etching/ablation patterns on a solid sample where the laser is hitting, you can see a degradation film/precipitate form from an aqueous sample, etc.
- You notice the Raman peak location changing over time – If you start to see the peak shift to lower frequencies, this is a strong indication your sample is being heated. The heating causes the sample to exist in an excited vibrational state, thus forcing a red shift in the Raman signal.
- You notice a broadening in your Raman peak width – This frequency broadening is due to heating of the sample as well. If the sample is being heated, the vibrational levels in the ground electronic state will be populated more evenly (as opposed to just the ground vibrational state, n = 1, being populated). Thus the initial laser excitation will from many different levels instead of just one, resulting in a broader Raman signal.
- You begin to see a large background in your spectrum – Raman spectroscopy is a scattering process and therefore has a great deal of angular dependence. If a solid sample is being damaged for instance, the surface may no longer be smooth on a microscopic level. This will cause a different scattering pattern which will oftentimes result in a large background signal.
- The Raman spectrum is changing drastically (2 peaks becoming 1, 1 peak becoming 3, etc.) – Since we are dealing with high power densities with laser sources, it is quite possible to induce chemical reactions or even phase transitions. For example, if one were studying the Raman spectrum of ice and didn’t account for the laser melting of the sample, one would expect to see the Raman spectrum transition from ice to that of liquid water.
Sample damage is always a consideration in laser spectroscopy, and is particularly important in Raman spectroscopy due to the combination of electronic and vibrational excitations. Always choose your laser source carefully, taking into consideration wavelength, pulse duration (if using a pulsed laser source), and power incident on the sample. While it is tempting to just fire the highest laser power possible at the sample to get the most signal out, this can be a kiss of death and will usually result in ruining that sample. Be sure to slowly ramp up the laser power until you see signs of damaging, then tune it back down below the damage threshold.
Citations:
(1) Deng, H.; Callender, R. Raman Difference Studies of Protein–Nucleic Acid Interactions. J. Raman Spectrosc. 1999, 30, 685–691.
(2) Vandenabeele, P.; Edwards, H. G. M.; Moens, L. A Decade of Raman Spectroscopy in Art and Archaeology. Chem. Rev. 2007, 107
(3), 675–686 DOI: 10.1021/cr068036i.
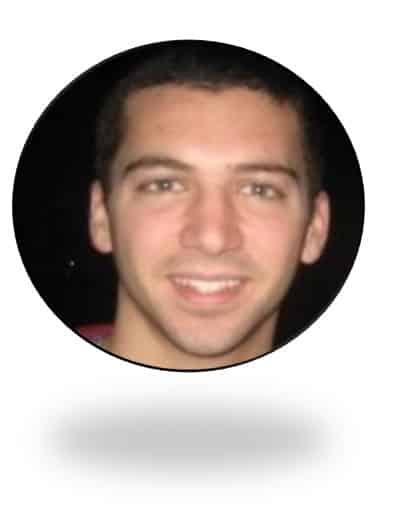
By Tony Rizzuto, PhD
StellarNet Technical Staff Writer
UC Berkeley Chemistry