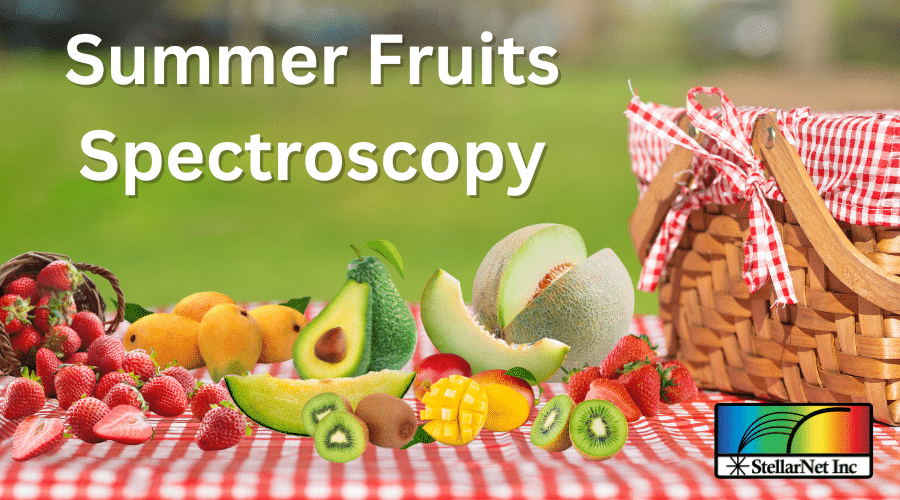
Calibration of the Components
Introduction
Fruits, as complex biological entities, embody a diverse array of components crucial for determining their quality. These components, varying across fruit types and even within species, encompass attributes such as firmness, sugar levels, water content, and acidity.
Traditionally, assessing these components necessitated destructive sampling methods, such as squeezing whole fruits directly into refractometers. However, such methods are not only invasive but also limit comprehensive quality control measures across batches due to the variability inherent in fruit composition.
Our objective centers on establishing correlations between spectrometer readings and key fruit components. By subjecting fruit samples from these varieties to spectrometer readings alongside conventional tests, we aim to uncover potential relationships between the two datasets. The overarching goal is to develop a calibration enabling the non-invasive determination of fruit component values using spectrometry, thus circumventing the need for destructive sampling.
Subsequent data analysis, employing a partial least squares regression (PLS) algorithm, aims to quantify the calibration’s performance, leveraging metrics like R² and RMSE. Through rigorous calibration and validation, our endeavor is to fortify spectrometry as an indispensable tool for precise fruit quality assessment and enhanced quality control practices.
Results
The table below presents the experimental values obtained from the study alongside average values sourced from literature.
Fruit | Brix (%) | Dry matter (%) | |
Honey Mango | Experimental value | Average: 16.20-23.20 Average: 20.31 |
Range: 16.99-27.04 Average: 23.14 |
Literature value | Range: 16.0-20.3 | Average: 16.9-20 | |
Honeydew |
Experimental value | Average: 8.1-10.1 Average: 9.21 |
Range: 5.23-11.75 Average: 7.65 |
Literature value | Minimum standard: 10 | Average: 10 | |
Kiwi |
Experimental value | Average: 9.10-14.9 Average: 13.09 |
Range: 12.03-21.08 Average: 16.31 |
Literature value | Minimum standard: 6.5 | Accepted standard: 15-20 |
Experimental results generally align with the literature, albeit with some minor discrepancies. The wide range of fruit component values underscores the complex interaction of numerous factors influencing fruit composition. Differences between experimental and literature values stem from factors like using different fruit cultivars, as well as external environmental conditions such as climate, humidity, and fertilization methods. These factors, combined with genetic variations, can lead to significant fluctuations in fruit components across different samples and studies.
Understanding these influences is crucial for accurately interpreting data. While literature values offer useful benchmarks, their applicability may be limited due to agricultural and environmental variability. Thus, a nuanced understanding of these factors is vital for interpreting experimental data and advancing our knowledge of fruit composition dynamics.
Below is a table showing the Brix and Dry Matter calibration results. R² and RMSE data are used to evaluate the performance of the calibration. R² (coefficient of determination) is a measurement between 0 and 1 that indicates how well the independent variables in the calibration explain the variability in the dependent variable. For example, an R² value of 0.70 means that 70% of the variability in the dependent variable is accounted for by the independent variables.
The closer the R² value is to 1, the more accurate the calibration. RMSE (Root Mean Square Error) measures the average magnitude of the prediction errors, indicating how much the actual values deviate from the predicted values. For instance, an RMSE value of 0.3 with a predicted value of 7 means that the actual value is likely between 6.7 and 7.3. In short, high R² values and low RMSE values indicate a more accurate and reliable calibration.
Fruit | Brix | Dry matter | |
Honey Mango | R² | 0.945 | 0.924 |
RMSE | 0.43 | 0.338 | |
Honeydew |
R² | 0.95 | 0.873 |
RMSE | 0.157 | 0.332 | |
Kiwi |
R² | 0.86 | 0.857 |
RMSE | 0.254 | 0.52 |
Mango Spectroscopy Results
The results of the PLS predictor for mango components demonstrate a high degree of predictive accuracy. With R² values of 0.945 for Brix and 0.924 for Dry Matter, the calibration explains 94.5% and 92.4% of the variance in these components, respectively, indicating a strong fit. Furthermore, the low RMSE values of 0.43 for Brix and 0.338 for Dry Matter underscore the calibration’s precision in predictions.
These findings suggest that the predictor is highly effective and reliable for determining the Brix and Dry Matter content in mangoes, making it a valuable tool for applications requiring accurate assessment of these fruit quality parameters.
However, there are potential limitations and areas for improvement. Since the calibration was done only with Honey mangoes in Florida, the current calibration may not fully capture the variability in other mango varieties and growing conditions. Including a more diverse range of mango types and environmental factors such as soil type, climate, and agricultural practices in the training dataset could enhance the calibration’s generalizability and robustness.
Honeydew Spectroscopy Results
Prediction using a spectrometer (absorbance spectra) is crucial for this experiment due to the intricate composition of honeydew, which includes sugars, organic acids, and amino acids, all contributing uniquely to its flavor and nutrition. Spectrometry offers a non-destructive method to analyze these components, providing detailed absorbance spectra that allow for precise quantitative assessment.
Despite the challenge of a limited sample size, the model successfully predicted important parameters such as Brix (R² = 0.95, RMSE = 0.157) and dry matter (R² = 0.873, RMSE = 0.332). Expanding the diversity of honeydew samples used for training would further refine the model’s accuracy, similar to advances observed in studies encompassing broader fruit varieties. The spectrometer’s ability to interpret these spectral signatures ensures exact measurement, thereby supporting dependable quality control and enabling informed decisions in agricultural and market settings where precise characterization of honeydew is indispensable for optimal utilization and commercial success.
Kiwi Spectroscopy Results
Predicting components like Brix and dry matter in kiwifruit poses unique challenges due to differences in maturity stages among varieties, which significantly affect their content. Achieving accurate predictions requires careful timing during harvest to maintain fruit quality. Additionally, kiwi’s complex internal structure complicates absorbance spectra analysis, unlike fruits such as honey mango and honeydew, which have smoother textures and simpler modeling processes.
Despite these challenges, the analysis of kiwifruit’s Brix and dry matter content, using absorbance spectra trained with a PLS model, demonstrates significant strides in predictive accuracy. The Brix measurement shows a strong correlation (R² = 0.86) with the absorbance spectra and PLS model, indicating that 86% of the variability in Brix values can be explained by the model. This demonstrates reliable estimation of sweetness. The RMSE of 0.254 reflects the average magnitude of prediction errors, showing the model’s accuracy in predicting Brix content. Similarly, for dry matter, the model performs well with an R² of 0.857, explaining 85.7% of the variability in dry matter values. The RMSE of 0.52 for dry matter indicates reasonable accuracy in predicting texture and nutritional density. These findings demonstrate the spectrometer’s ability to accurately predict kiwi components, essential for meeting consumer preferences and industry standards.
Testing Method
Materials
Strawberries, avocados, kiwi, honey mango, honeydew melon, cutting board, knife, distilled water, beakers, pH meter, Atago refractometer, Atago acidity meter, pH probe, firmness penetrometer, pipettes, analytical scale, weigh boats, dehydrator, aluminum foil, lemon juicer, core cutter.
Spectrometry
For each fruit, its flattest side (2-8 sides depending on fruit size) that covered all the light from the fixture was positioned onto the fixture of a UV-VIS spectrometer. Multiple readings (typically 2-3) were taken from each labeled side to ensure representative data collection. This process was systematically repeated for all labeled sides of the fruit to cover its entire surface area. The UV-VIS spectrometer was configured to measure absorbance spectra across a wavelength range of 340 to 1170 nm, enabling comprehensive characterization of the fruit’s spectral properties.
Firmness
The firmness of the fruit was assessed using a fruit penetrometer. To ensure precision, each fruit underwent three measurements using the penetrometer. These individual readings were meticulously recorded, and their collective average was calculated. This average value served as the basis for calibration.
Dry Matter
After conducting the firmness test, a small fruit (such as a strawberry or kiwi) was precisely sectioned along either its sagittal or coronal plane to obtain a thin sample weighing approximately 3-5 grams. For larger fruits like honey mangoes, honeydew melons, and avocados, a circular core cutter was employed to extract a sample from the region where spectroscopic readings were taken. The extracted fruit samples were then placed in a dehydrator set at 62.8°C for a duration of 24 hours.
Subsequently, the weight of each dehydrated fruit slice was measured, followed by another 24-hour period in the dehydrator. After a total dehydration time of 48 hours, the weight of the strawberry slices was measured once more. The lowest of the two values were taken and the formula [(Wi–Wf)/Wi] x 100 where Wi is the initial weight and the Wf is the final weight was used to determine the moisture percent in the strawberry. Subtract the moisture percent from 100 to get the dry matter %.
Brix
The remaining portion of the fruit sample (excluding the 3-5g portion designated for dehydration) underwent juicing using a lemon juicer, with the extracted juice filtered through a coffee filter and collected in a small cup lined with aluminum foil. After thorough stirring, 0.5mL of the strawberry juice was carefully pipetted onto the surface of a refractometer. The resulting Brix reading was then recorded.